RESOLUTION- AND THROUGHPUT-ENHANCED SPECTROSCOPY USING HIGH-THROUGHPUT COMPUTATIONAL SLIT
There exists a fundamental tradeoff between spectral resolution and the efficiency or throughput for all optical spectrometers. The primary factors affecting the spectral resolution and throughput of an optical spectrometer are the size of the entrance aperture and the optical power of the focusing element. Thus far collective optimization of the above mentioned has proven difficult. Here, we introduce the concept of high-throughput computational slits (HTCS) for improving both the effective spectral resolution and efficiency of a spectrometer. The proposed HTCS approach was experimentally validated using an optical spectrometer configured with a 200 um entrance aperture (test), and a 50 um entrance aperture (control), demonstrating improvements in spectral resolution of the spectrum by ~20% over the control spectral resolution and improvements in efficiency of > 2 times the efficiency of the largest entrance aperture used in the study while producing highly accurate spectra.
For more information on high-throughput computational slits (HTCS), please read:
1. F. Kazemzadeh and A. Wong, Resolution- and throughput-enhanced spectroscopy using high-throughput computational slit. Optics Letters, 2016.
2. E. Kuang, F. Kazemzadeh, and A. Wong, Enhanced Smartphone Spectroscopy via High-throughput Computational Slit. Journal of Coputational Vison and Imaging Systems, 2016.
3. A. Wong and F. Kazemzadeh, A System, Method, and Apparatus for Improving the Spectral Resolution and Signal-to-Noise Ratio of Optical Spectrometer Using Digital Beam Refocusing, Reforming, Slicing, and Multiplexing. United States Patent Application 62/199,732.
Overview of high-throughput computational slit (HTCS). The systems design of a generic spectrometer is shown here augmented with the proposed HTCS. Pictorial representation of the beam shape demonstrate how the HTCS system operates by changing the morphology of light that has entered from a much larger entrance aperture (a)to increase the spectral resolution of the spectrometer by imposing a small-slit function(b). (c) The high-resolution spectrum of the light source used for this study, Mercury vapor fluorescent lamp captured using a 10 µm entrance aperture. (d) Shows the imaged spot morphology after the light propagates through a normal aperture. (e) Shows the imaged spot morphology after the light propagates through the proposed HTCS. (f) The spectra of the light source at one second exposure time using a 200 µm and a 50 µm entrance aperture used for light throughput comparison. It is noted at the marked wavelength of interest, 613 nm, the peak signal is > 14 times larger using the larger entrance aperture. (g) The normalized spectra of the source using a 200 µm and a 50 µ m entrance aperture used for spectral resolution comparison. It is noted at at the marked wavelength of interest, 438 nm, the spectral resolution is > 4 times higher using the smaller entrance aperture.
(h) The spectra of the light source at one second exposure time using a 200 µm and a 50 µm entrance aperture and the HTCS implementation on the 200 µm entrance aperture. At wavelength of interest, 613 nm, the peak signal of the spectrum produced by the HTCS method is > 2 times and > 30 times larger than the spectrum of 200 µm and a 50 µm entrance aperture, respectively. (i) The spectra of the 200 µm and a 50 µm entrance aperture and the HTCS implementation on the 200 µm entrance aperture, normalized at 546 nm, which is a doublet. It can be observed that the spectral features from the HTCS implementation closely resembles the the features observable in the 50 µm spectrum.
There exists a fundamental tradeoff between spectral resolution and the efficiency or throughput for all optical spectrometers. The primary factors affecting the spectral resolution and throughput of an optical spectrometer are the size of the entrance aperture and the optical power of the focusing element. Thus far collective optimization of the above mentioned has proven difficult. Here, we introduce the concept of high-throughput computational slits (HTCS) for improving both the effective spectral resolution and efficiency of a spectrometer. The proposed HTCS approach was experimentally validated using an optical spectrometer configured with a 200 um entrance aperture (test), and a 50 um entrance aperture (control), demonstrating improvements in spectral resolution of the spectrum by ~20% over the control spectral resolution and improvements in efficiency of > 2 times the efficiency of the largest entrance aperture used in the study while producing highly accurate spectra.
For more information on high-throughput computational slits (HTCS), please read:
1. F. Kazemzadeh and A. Wong, Resolution- and throughput-enhanced spectroscopy using high-throughput computational slit. Optics Letters, 2016.
2. E. Kuang, F. Kazemzadeh, and A. Wong, Enhanced Smartphone Spectroscopy via High-throughput Computational Slit. Journal of Coputational Vison and Imaging Systems, 2016.
3. A. Wong and F. Kazemzadeh, A System, Method, and Apparatus for Improving the Spectral Resolution and Signal-to-Noise Ratio of Optical Spectrometer Using Digital Beam Refocusing, Reforming, Slicing, and Multiplexing. United States Patent Application 62/199,732.
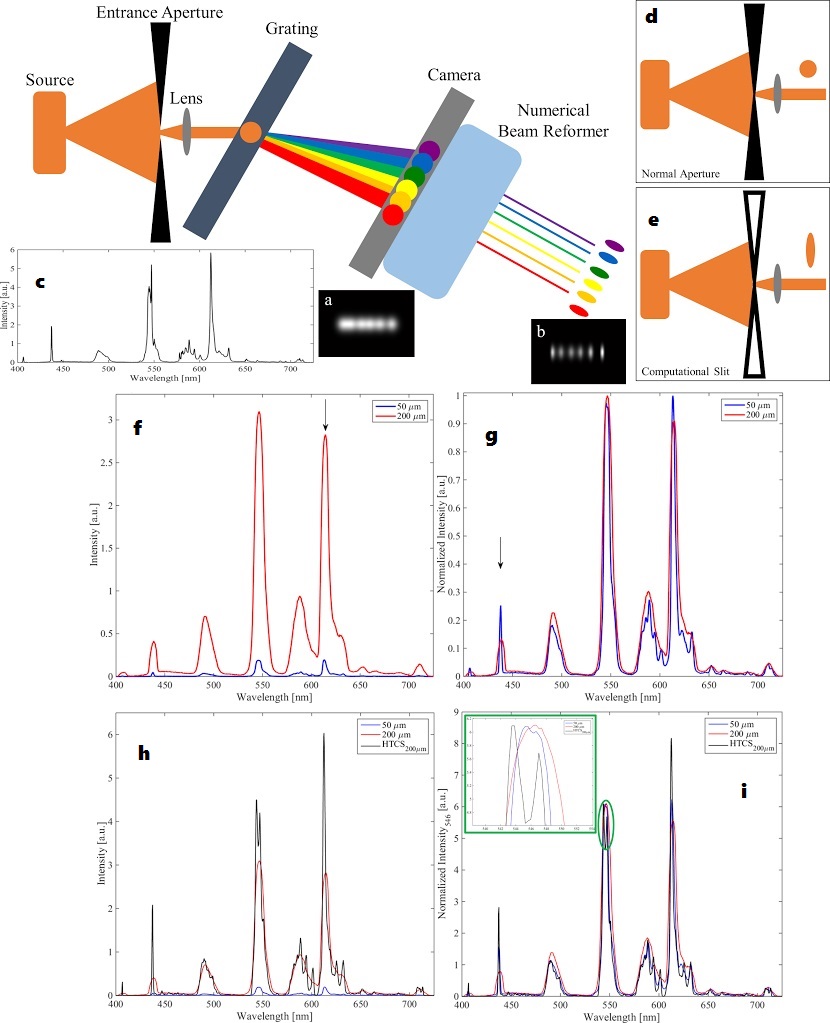
Overview of high-throughput computational slit (HTCS). The systems design of a generic spectrometer is shown here augmented with the proposed HTCS. Pictorial representation of the beam shape demonstrate how the HTCS system operates by changing the morphology of light that has entered from a much larger entrance aperture (a)to increase the spectral resolution of the spectrometer by imposing a small-slit function(b). (c) The high-resolution spectrum of the light source used for this study, Mercury vapor fluorescent lamp captured using a 10 µm entrance aperture. (d) Shows the imaged spot morphology after the light propagates through a normal aperture. (e) Shows the imaged spot morphology after the light propagates through the proposed HTCS. (f) The spectra of the light source at one second exposure time using a 200 µm and a 50 µm entrance aperture used for light throughput comparison. It is noted at the marked wavelength of interest, 613 nm, the peak signal is > 14 times larger using the larger entrance aperture. (g) The normalized spectra of the source using a 200 µm and a 50 µ m entrance aperture used for spectral resolution comparison. It is noted at at the marked wavelength of interest, 438 nm, the spectral resolution is > 4 times higher using the smaller entrance aperture.
(h) The spectra of the light source at one second exposure time using a 200 µm and a 50 µm entrance aperture and the HTCS implementation on the 200 µm entrance aperture. At wavelength of interest, 613 nm, the peak signal of the spectrum produced by the HTCS method is > 2 times and > 30 times larger than the spectrum of 200 µm and a 50 µm entrance aperture, respectively. (i) The spectra of the 200 µm and a 50 µm entrance aperture and the HTCS implementation on the 200 µm entrance aperture, normalized at 546 nm, which is a doublet. It can be observed that the spectral features from the HTCS implementation closely resembles the the features observable in the 50 µm spectrum.